Around 13.7 billion years ago, something collapsed. It fell outward into the nothingness that stretched in every direction, leaving something behind. A dense plasma, made of fundamental particles called quarks and gluons. For one tiny moment, the plasma collapsed outward unimaginably fast. Then, reigned in by the quarks coalescing into matter, the collapse began to coast.
The universe cooled. Small clumps of matter, set by random chance in the first moments of its existence, attracted more matter through gravity. They became gas clouds, and then galaxies. Galaxies formed galaxy clusters. And empty space continued to expand.
This was the Big Bang, and it set off the expansion of the universe. Today, we’ll look at the strange forces that drive the acceleration of its expansion, as well as one of the most tantalizing open questions in modern astronomy: Exactly how fast is it expanding today? And why can’t we figure that out?
The expanding universe
In 1929, an astronomer named Edwin Hubble found that every single galaxy outside of the Local Group — our neighbors — was speeding away from us. Galaxies like Andromeda, our nearest large neighbor, had stars that looked more or less the same color as the ones in the Milky Way. But beyond that, every galaxy looked red.
This, Hubble reasoned, was because of the Doppler effect. When a fire truck is driving away, its siren sounds like a decrescendo because the outgoing velocity stretches out the sound waves. The chances of every galaxy outside our local sphere of the universe being naturally red were small. Hubble concluded the simplest explanation was that every galaxy was moving away from us.
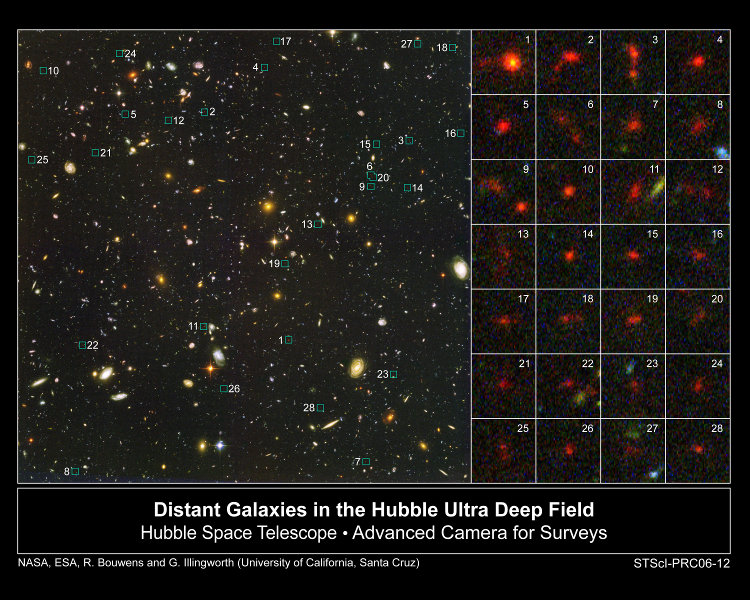
More distant galaxies appear redder due to the Doppler effect. Photo: NASA/ESA/Bouwens/Illingsworth
At first glance, this implies there is something special about the Earth. But one of the principles of cosmology, the study of the birth, death, and overall structure of the universe, is that there are no special locations. Any galaxy perceives all distant galaxies as moving away. This is because the space in between galaxies is expanding with every passing second.
In 1998, a team of cosmologists observed the recession velocities of distant galaxies and noticed something strange. At very high distances, galaxies were even further away than they should have been if the velocity was constant. That meant the universe hadn’t expanded at a constant rate.
Instead, it’s accelerating over time.
Introducing dark energy
According to Newton’s first law, objects in motion will stay in motion unless acted on by an outside force. Once galaxies were moving apart from one another due to the Big Bang, therefore, it made sense for them to continue on their trajectories. But for the universe to be accelerating meant that a force was driving that acceleration.
Astronomers dubbed this new factor dark energy, because they didn’t know what it was. It’s still perplexing, but in the decades since, dark energy has become accepted as part of the universe’s source code. Mass attracts mass, like-magnets repel one another, and empty space naturally expands.
Dark energy says: you can’t have a true vacuum. Seemingly empty space actually has natural energy, and it wants to get out. It expands — and if it’s already expanding, that expansion accelerates.
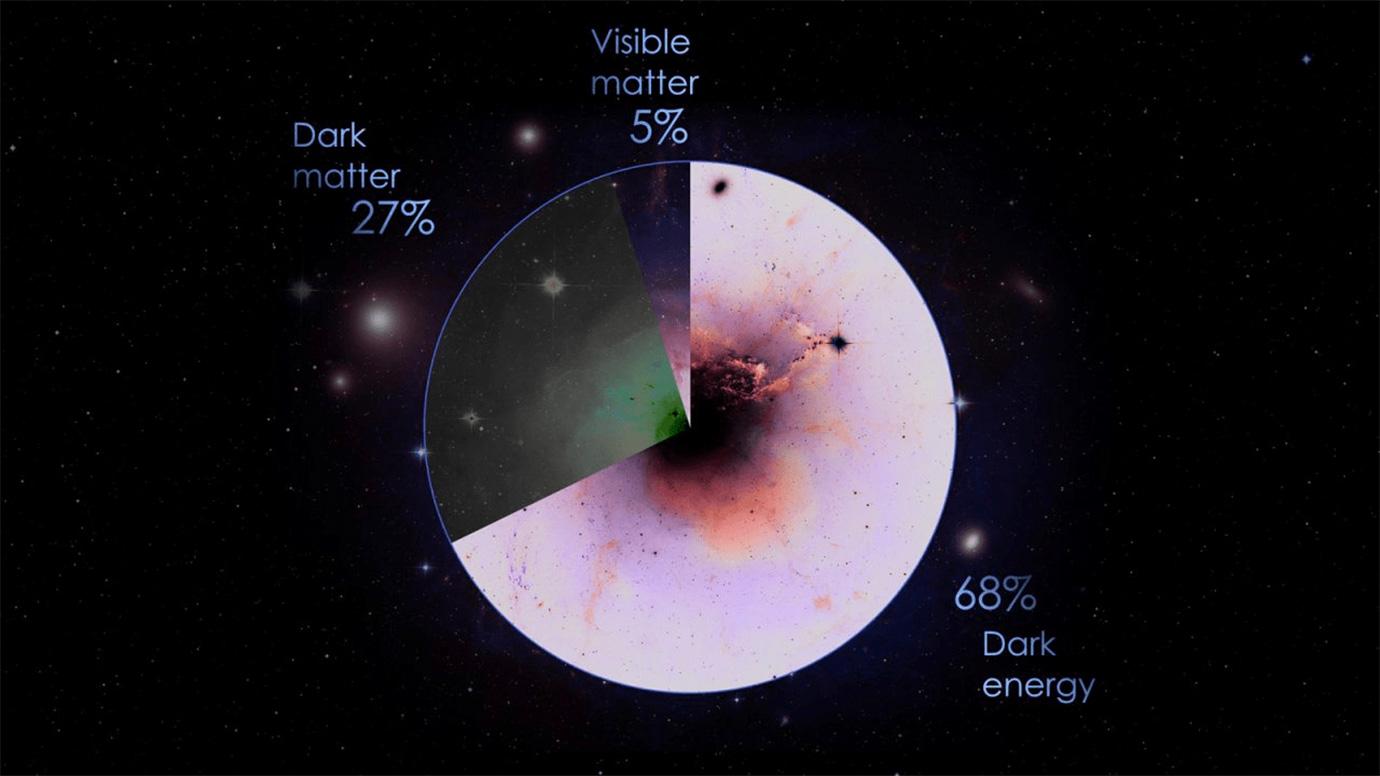
As shown in Einstein’s famous equation E = mc^2, energy and mass are convertible. Dark energy accounts for a huge part of the universe’s mass. Photo: NASA
The Hubble Tension
So if the expansion velocity of the universe isn’t always the same, what is its present value?
For modern cosmologists, the answer to that question is a Holy Grail. It’s not that we have no idea. We have too many ideas. Over and over again, the same two values keep cropping up: 67 km/s and 73 km/s.
In the past, astronomers like Edwin Hubble didn’t realize the expansion was accelerating because their observations didn’t reach far enough, and when they did, they weren’t very precise. But over the last two decades, astronomers have developed very sophisticated techniques for measuring the expansion of the universe. About half of those techniques give a modern expansion velocity of 67 km/s, and the other half give 73 km/s.
At first, cosmologists assumed the values differed because the measurements weren’t precise enough. The error bars covered the whole range of values between the two limits, so there was nothing to worry about.
Then the measurements got better and better, the error bars shrank, and still the two values stayed separate. Why?
Cosmic Microwave Background
The further away we look, the further back in time we look. That’s because light takes time to travel toward us, so the light from very distant parts of the universe reaches us billions of years after it was emitted. As we look back, we see modern galaxies, then middle-aged ones, and then the strange clumpy squiggles of very ancient galaxies. Then we see the wall.
It’s not a physical wall. Instead, it’s the image of a time when everything in the universe was on fire. It’s called the Cosmic Microwave Background, or CMB. It formed when protons and electrons merged into atoms, suddenly releasing the photons from ricocheting around the primordial plasma. Those photons have been traveling ever since, and are reaching us every day. Part of the static on any radio station or television screen comes from the earliest observable light in the universe.
The CMB isn’t perfectly identical in every direction. It has clumps and divots, and those ancient clumps and divots laid the blueprint for the distribution of galaxies in the modern universe.
Cosmologists plug the attributes of those clumps into a general relativity model of the universe starting at the Big Bang. When the clock reaches the present day, the universe is expanding at about 67 km/s.
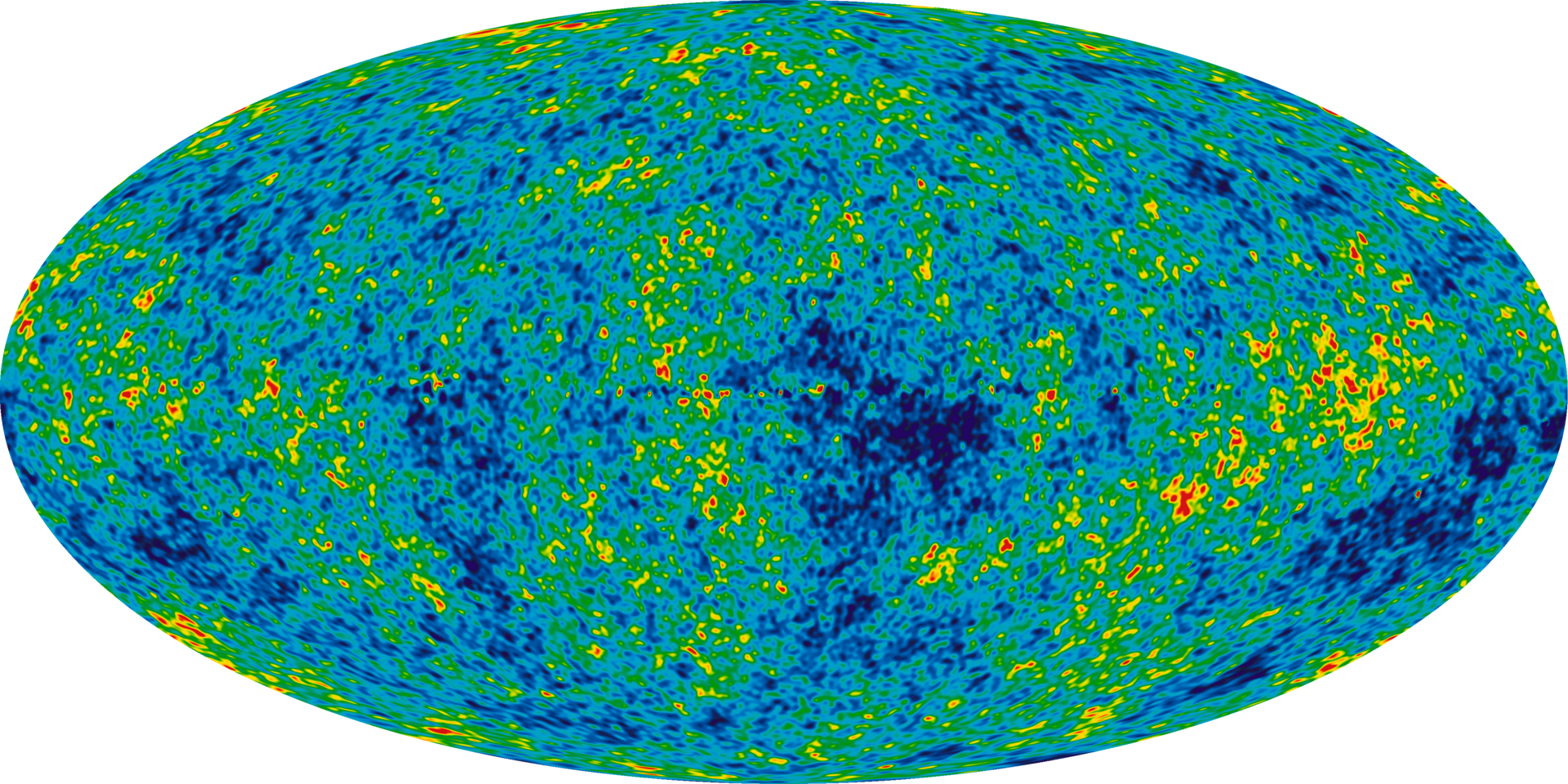
This famous map of the Cosmic Microwave Background is deceptive. In fact, the visual differences are magnified by ten thousand times their actual strength. Photo: WMAP/NASA
Wobbling Cepheids
So far, general relativity has withstood every test that’s come its way. Why is it, then, that its predictions for the current expansion velocity from the CMB don’t match our observations from galaxies?
Figuring out the current velocity from galaxies requires knowing how far away those galaxies actually are. Astronomers use something called the cosmic distance ladder to connect observations within our own galaxy to observations in other galaxies.
First, they observe nearby stars called Cepheids. Cepheids don’t have a constant brightness. Instead, they get periodically dimmer before brightening again. The brighter a Cepheid is, the faster its light fluctuates. By observing how fast they fluctuate, astronomers can calculate how bright a Cepheid in another galaxy really is, instead of just how bright it looks when viewed from far away. That gives the distance to the galaxy.
Every so often, two orbiting white dwarfs in these galaxies collide, leading to a massive explosion called a Type 1a supernova (supernova astronomers are infamous for their opaque naming conventions).
Using the known distance of the galaxies, astronomers figured out that the peak brightness of these supernovae controls how fast the light fades. So if a Type 1a supernova goes off in a distant galaxy, we know from the light decay speed how bright it should appear. Once again, we can extrapolate the distance of the galaxy based off how bright its most exotic stars actually appear.
Finally, the Doppler effect allows us to calculate recession velocity of those galaxies. It’s always about 73 km/s.
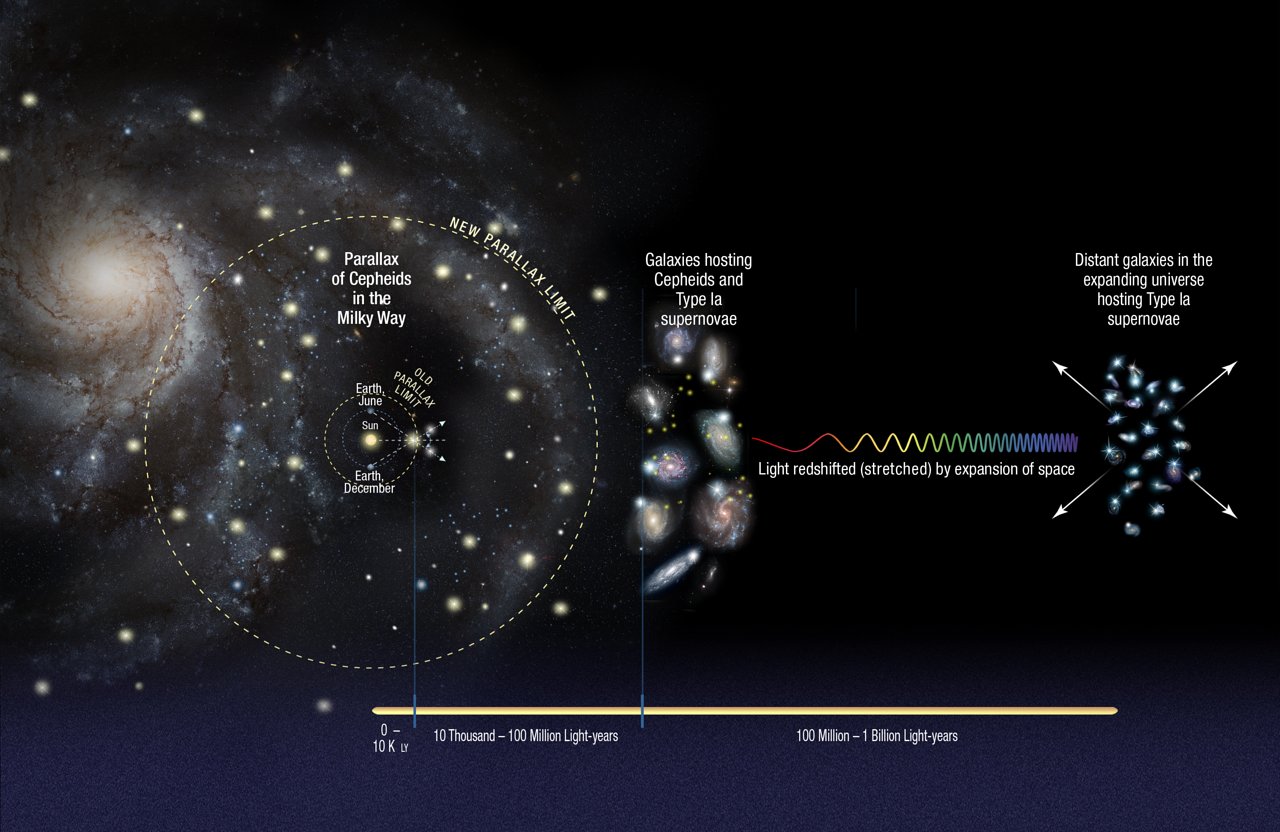
The cosmic distance ladder allows astronomers to find the distance of nearby to distant galaxies. Photo: ESA/Hubble
What causes the Hubble Tension?
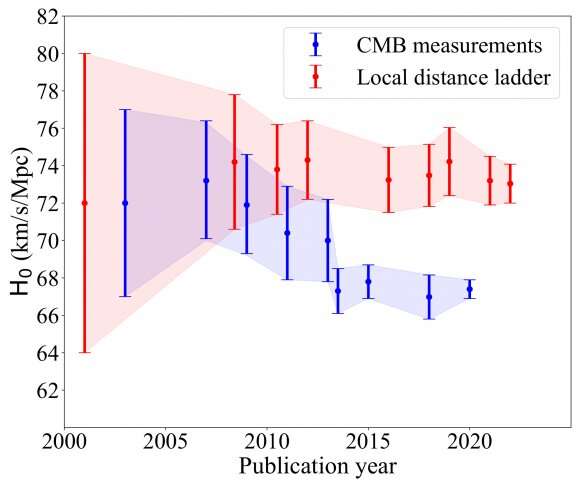
Over time, the two methods of determining the expansion velocity have diverged. Photo: Perivolaropoulos and Skara 2022
The discrepancy between these two measurements is called the Hubble Tension, and it doesn’t seem to be going away. Other methods of calculating distance from galaxies — microwave lasers and neutron star mergers, for instance — give the same result as the supernovae of 73 km/s. Meanwhile, no matter what methods are used to calculate the expansion velocity from the CMB, they all give 67 km/s. A handful of measurements fall somewhere in between, but the vast majority pick a side and stick to it even as their error bars shrink.
It’s not clear what’s going on. The universe can’t be expanding at both 67 km/s and 73 km/s at the same time, so we’re going wrong somewhere. Is there some recurring error in how astronomers are calculating distance of galaxies? Are the CMB measurements not as accurate as we think?
Why don’t observations in the recent, galaxy-inhabited universe give us the same answers as observations from the very beginnings of the universe. And which of the answers is correct?
Anyone who can answer that question is practically guaranteed a Nobel Prize. But right now, cosmologists have no idea.
The dominant theory is that dark energy was stronger in the early universe in a way the models don’t take into account. That would mean that no matter how precise our CMB measurements are, our models aren’t correctly extrapolating that information to the present day. But if that were the case, why would dark energy change over time? Isn’t it supposed to be a constant of the universe?
There are other, more outlandish theories involving strange “dark” photons that have pushed the modern universe into an increased expansion velocity. But the most disconcerting prospect is not the existence of yet another strange physical substance with “dark” in its name: it’s the idea that our model of gravity itself is somehow fundamentally flawed. If so, the story of the universe will have to be rewritten.